Review Article
Recent Advancement in the Formulation and Evaluation of Micro Particle and its Application
2760
Views & Citations1760
Likes & Shares
Introduction: The micro particles and development in the area of micro particles analysis are discussed in this article.
Areas covered: Micro particles are a unique drug carrier method that provides an effective therapeutic alternative to single-unit dose forms that are either traditional or rapid release. Produce the micro particles by filling them with firm gelatin or compressing them immediately. When compared to traditional dosage forms, micro particles manufactured using various types of technologies vary their performance and administration of the dosage form.
Micro particles have been tested using in vitro release techniques like dialysis membrane sacs, Sample and separate and USP equipment IV. According to comparisons of these techniques, USP apparatus IV is the preferred method right now. Accelerated in vitro release assays were created to reduce the amount of time required for quality control testing. To reduce the necessity for in vivo performance analysis, in vitro-in vivo correlation using real-time and accelerated release data have been produced. Storage stability studies have been carried out to see how different environmental conditions affect microsphere quality over the course of the product's life span (t90). New tests like the in vitro wash off test and floating test have been introduced, as have characterization approaches for various physico-chemical characteristics such drug content, thermal properties and particle size.
Keywords: Micro particles, Polymer, Clarithromycin, Micrometrics, Antibiotic, Orthopedic, Microcapsules, USP apparatus IV
INTRODUCTION
A regulated pharmaceutical delivery system can help address a few of the drawbacks of traditional therapy while simultaneously boosting therapeutic effects. To achieve maximum therapeutic efficacy, the chemical must be transported to the correct location in the appropriate amount and at the correct time, with the least degree of toxic effect as possible. A therapeutic substance can be given to the target area in a number of controlled and consistent methods. A few of these ideas is to use micro particles as medication carriers [1].Micro particles are free-flowing spherical powders composed of synthetic, biodegradable and non-bio degradable polymers with a particle size should be between 1 to 1000 microns shown in Figure 1. The main purpose of a revolutionary drug delivery system like this is to bypass the limits of traditional dosage forms by boosting increasing bioavailability, patient compliance and more precisely targeting medicines or other active substances [2]. There are two types of micro particles;
• Microcapsules.
• Micrometrics [2].
Microcapsules have a recognizable capsule wall around the encapsulated material, whereas micro rays have the encapsulated ingredient dispersed within the particulate matrix with the potential for controlled release [3].
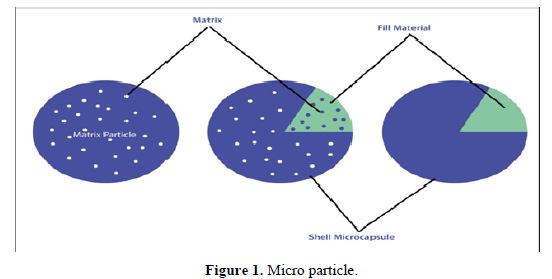
Advantages of Micro particles
- Decrease of the size contributes to an increasing the area of the surface and can increase the potency of a material that is difficult to dissolve.
- Providing a steady quantity of medications in the body that can improve patient compliance.
- Dose and risk reduced.
- Drug packaging with polymers prevents the drug avoid enzymatic cleavage while making it suitable for drug method delivery system.
- Less duration of dosing contributes to higher patient compliance.
- Effective usage of medications can enhance bioavailability, and decrease harmful effects occurrence or severity.
- Helps protect the GIT from opioids irritants.
- Transform liquid into solid shape and block the unpleasant taste [4].
Disadvantages of Micro particles
- The pace of release of the regulated dose procedure, which varies depending on a number of parameters such as food and intestinal transfer levels.
- Changes in discharge rate from one dosage to the next.
- Because controlled release formulations have a larger dosage load, any flaws in the drug substance's release qualities can cause problems such as
- Potentially dangerous.
- These dosing types must not be broken or chewed [5].
Materials used in the micro particle formulation
They are classified as follows in the formulation of micro particle polymers:
- synthetic polymer
- Natural polymer
- Synthetic polymers are divided into two parts
- Non biodegradables polymers: Epoxy polymers, Poly methyl methacrylate and Acrolein glycidyl methacrylate.
- Biodegradables polymers: Glycosides, Lactides, and their co-polymers, Poly alkyl cyano-acrylates and Poly anhydrides.
TYPES OF MICRO PARTICLES
- Bio-adhesive micro particles
- Magnetic micro particles
- Radioactive micro particles
- Floating micro particles
- Polymeric Micro particles.
- Biodegradable polymeric micro particles.
- Synthetic polymeric micro particles [10].
Bio adhesive Micro particles
The capacity of a medication to attach to a membrane via the adhesive capabilities of aqueous-soluble polymers is known as adhesion. The adherence of a drug carrier to a mucous membrane, like the nasal mucosa, rectal, ocular or buccal is referred to as bio adhesion. These micro particles which are shown in Figure 2 spend more time at the application site, resulting in closer contact with the site of absorption and improved pharmacological action [11-13].
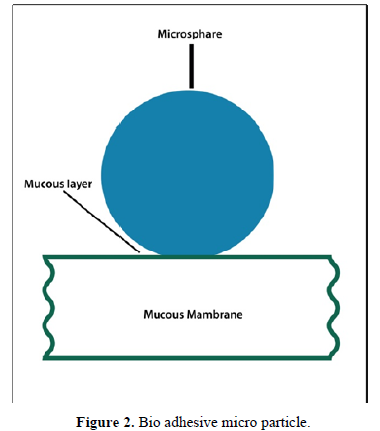
Magnetic Micro particles
The medicine is targeted to the illness spot using magnetic micro particles. Magnetic carriers collect magnetic responses from integrated materials and transmit them to the magnetic field. These magnetic micro particles shown in Figure 3 are made from chitosan, dextran, and other polymers. The magnetic micro particle has the advantage of allowing a large volume of freely spreading pharmaceuticals to be replaced with a smaller amount of magnetically focused meds [14].
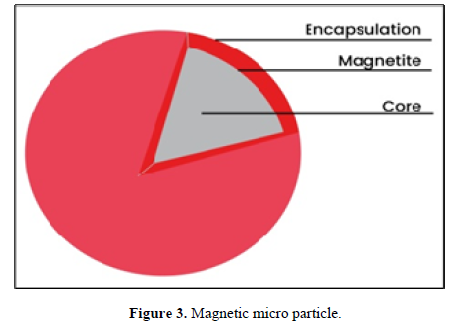
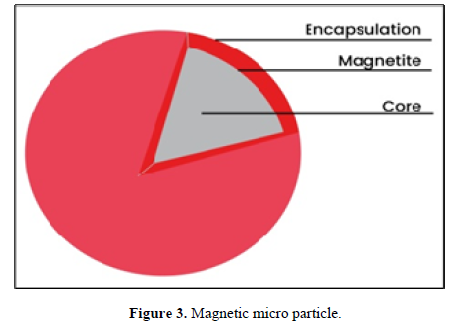
Radioactive Micro particles
Radiofrequency immobilization (RFI) is a therapy that uses radio waves to keep the patient immobilized. When micro particles with a diameter of 10-30 nm come into contact with the capillaries, they strike the first capillary bed. They are inserted into the arteries that supply the tumor with oxygen and nutrients. In all of these scenarios, radioactive micro particles shown in Figure 4 provide a large dose of radiation to target regions while inflicting no injury to nearby the healthy tissue. The various types of radioactive micro particles are called α-emitters, β-emitters, and γ-emitters [15,16].
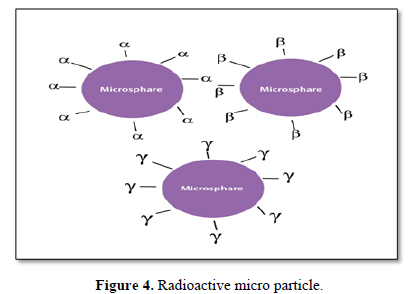
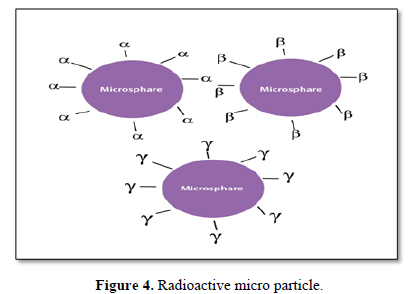
Floating Micro particles
The micro particle shown in Figure 5 floats in the stomach because it’s apparent density is less than that of stomach fluid. The drug is slowly released and at the ideal rate when the whole system is in motion with the gastric contents, which improves the residence in the stomach and the variability of the plasmatic concentration. This method has a longer-lasting therapeutic effect and reduces the number of doses required. With each successive stomach emptying, the sink particles will scatter throughout a vast absorption site, increasing the likelihood of a more or less predictable drug absorption and release profile. Also, because each dose is made up of numerous subunits, there is less chance of dose drift [17-19].
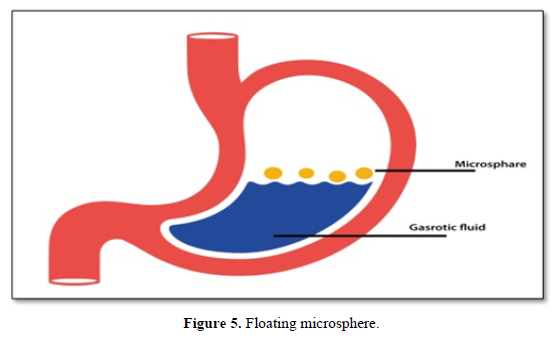
Polymeric Micro particles
Polymeric micro particles shown in Figure 6 are classified as follows:
Synthetic polymers
In medical applications, such as embolic particles, bulking substances, drug carriers, and other applications, synthetic polymeric micro particles have been proved to be secure and biocompatible. The major drawbacks of these micro particles are that they move away from the injection site, raising the risk of embolism and tissue injury [20].
Polymers that degrade biodegradable
Natural polymers, such like starch, are biodegradable, biocompatible and bio adhesive. Due to their high degree of swelling in the aqueous media, biodegradable polymers extend the residence duration whenever they come into contact with the mucosa, causing gels to develop. The rate and quantity of medicine released can be adjusted by gradually changing the polymer concentration and drug release profile. The main issue is that in medical applications, the drug loading capacity of biodegradable micro particles is challenging, making drug release difficult to handle [21].
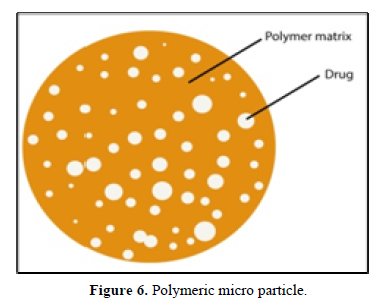
Method of Preparation
1. Spray drying
2. Solvent evaporation technology
3. Single emulsion technique
4. Double emulsion technique
5. Phase separation coacervation technology
6. Spray drying and Spray freezing
7. Solvent Extraction
8. Quasi-emulsion solvent diffusion.
Spray drying
The polymer is softened in a volatile organic solvent like acetone or dichloromethane and then the medication is homogenized in the polymer solution. The dispersion is subsequently atomized in a stream of hot air, generating small droplets through which the solvent quickly evaporates, resulting in micro particles with sizes ranging from 1 to 100 μm in diameter. A centrifugal separator separates the generated micro particles using hot air, and solvent residues are eliminated by vacuum drying shown in Figure 7 [22,23].
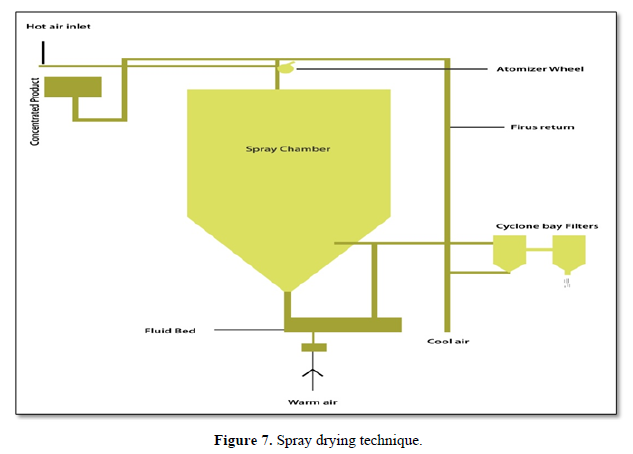
Solvent evaporation technology
It is one of the earliest methods of producing micro particles. An organic solvent, such like methylene chloride, must've been soluble in both the medication and the polymer. Drops can occur when a polymer and drug solution is dispersed in an aqueous medium. The more volatile organic phase can be evaporated with continuous mixing and increased temperatures, leaving the solid polymer-drug particles floating in aqueous solution. The suspension is then washed to remove the remaining particles. This procedure shown in Figure 8 [24].
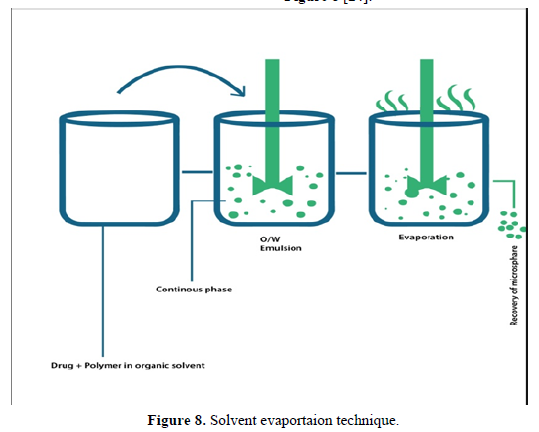
Single emulsion technique
A number of carbohydrate and protein products are produced using this technology. Natural polymers are soluble in an aqueous media and spread in a non-aqueous medium (oil phase) in this process, with the disseminated particles then cross-linked in one of two ways:
• By Heating: Dispersion in hot oil, nevertheless, for heat-labile medicines, this method is useless.
• Use a chemical cross-linking agent such as glutaraldehyde, formaldehyde or acid chloride, for example. Chemical crosslinking has a disadvantage: excessive exposure [24] (Figure 9).
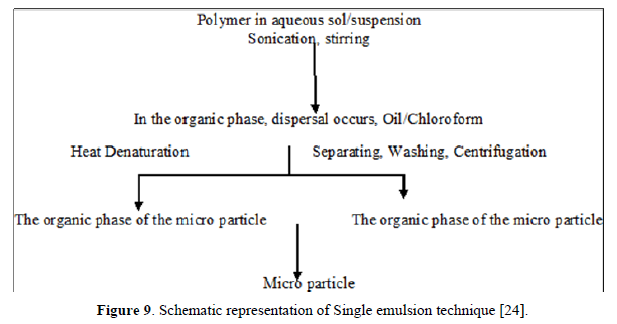
• Use a chemical cross-linking agent such as glutaraldehyde, formaldehyde or acid chloride, for example. Chemical crosslinking has a disadvantage: excessive exposure [24] (Figure 9).
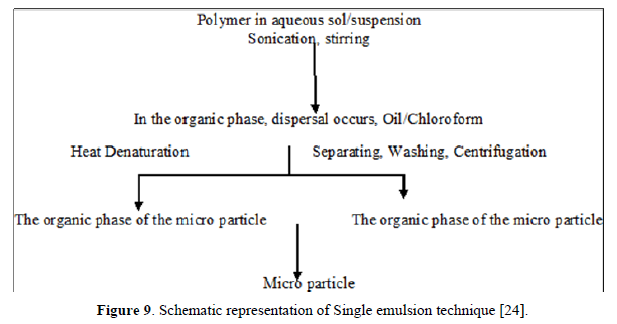
Double emulsion technique
Multiple emulsions or double emulsions of the w/o/w type are created in this micro particle production method, which is appropriate for water-soluble medicines, proteins, vaccines and peptides. This technique shown in Figure 10 can be used on both manufactured and natural polymers. Throughout the lipophilic organic continuous phase, the aqueous protein solution is dispersed. The active ingredients may be present in this protein solution [25-27].
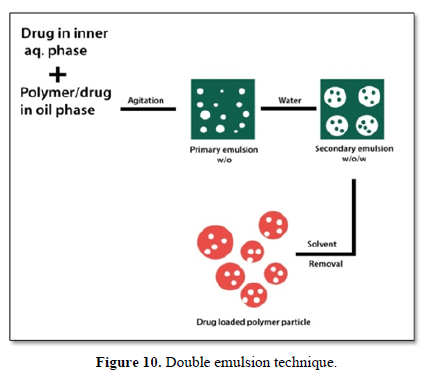
Phase separation coacervation technology
Phase separation method is commonly used to prepare the deposit kind of a system. This approach is used to enclose water-soluble pharmaceuticals including peptides and proteins, as well as some matrix-type formulations, especially when the medication is hydrophobic like steroids. The method is depended on reducing the solubility of the polymer in the organic phase to influence the formation of coacervates, a polymer rich phase. When a third component is added to the system, coacervation occurs, resulting in the creation of two phases, one with a lot of polymers and the other is not, i.e., supernatant, which is depleted in polymer. A variety of technologies can be used to phase separate coacervates. Techniques include salt addition, solvent addition, and incompatible polymer addition (Figure 11) [11,28,29].
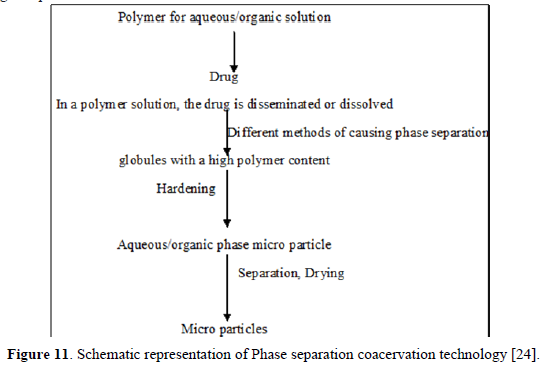
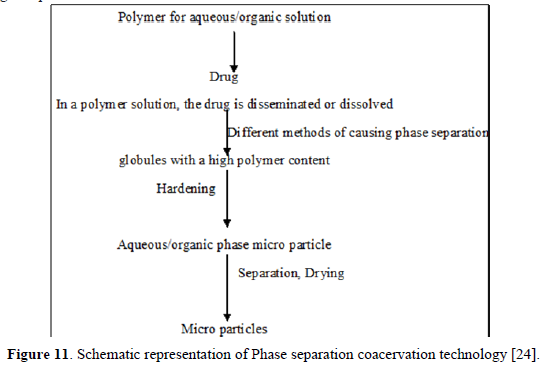
Spray drying and spray freezing-
These techniques shown in Figure 12 are based on a polymer and drug spray that are air dried. Spray drying and spray freezing are two processes that differ in that the solvent is removed or the solution is cooled [30,31].
Spray congealing: Spray = Hot melt/Cold air
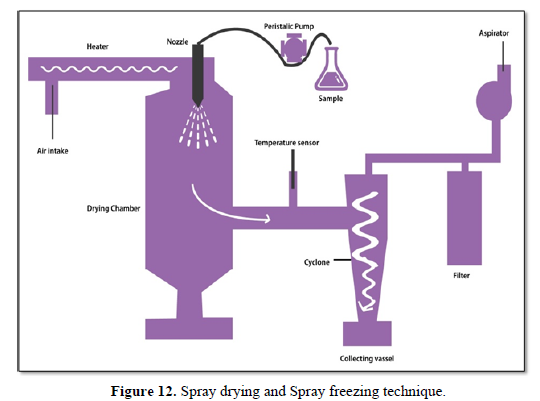
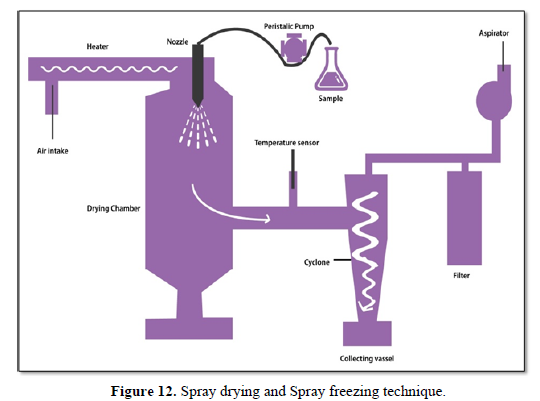
Extraction of solvents
The solvent evaporation method involves extracting the non-aqueous solvent and eliminating the organic phase to create micro particles. In this method, isopropanol is employed, which is a water miscible organic solvent (Figure 13) [32].
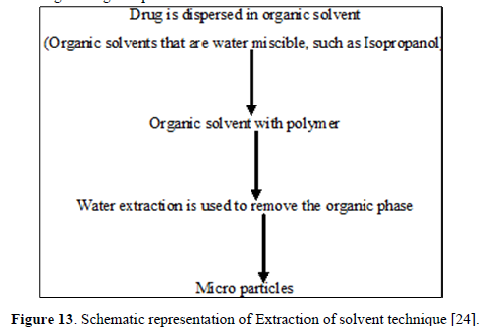
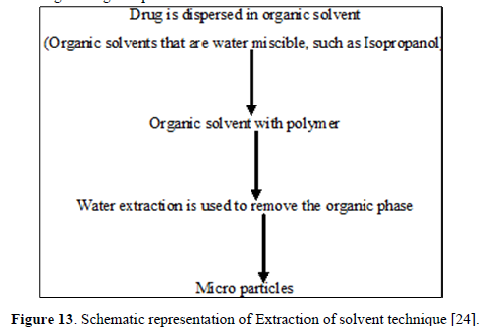
Quasi emulsion solvent diffusion
The literature has published a unique quasi-emulsion solvent diffusion approach to generate controlled-release drug-release micro particles with acrylic polymers. Quasi-emulsion solvent diffusion technique shown in Figure 14 which uses polyvinyl alcohol and purified water as the outer phase can be used to make micro sponges. In the internal phase are drugs, ethanol and polymers. The inner phase is initially produced at 60 degrees centigrade and then the outer phase is introduced at room temperature. The mixture is centrifuged indefinitely for two hours to emulsify it. By sieving the mixture, the micro sponges can be isolated from the rest [33,34].
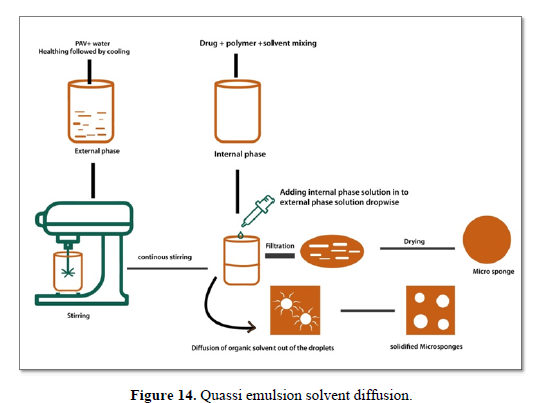
EVALUATION OF MICRO PARTICLES
Physicochemical evaluation
Particle shape and size
Traditional scanning light microscopy and electron microscopy are the most widely used technologies to observe micro particles. Either method can be used to identify the shape and exterior micro particle’s structure. In double-walled micro particles, optical microscopy allows precise control of coating conditions. The architecture of the micro particles can be seen before and after polishing and the modifications can be examined through microscopy. The resolution of (SEM) scanning electron microscopy is better than that of light microscopy. After the micro particles have been cross-sectioned, (SEM) scanning electron microscopy can be used to examine their surfaces, as well as double-wall systems. Using confocal fluorescence microscopy, the structure of many walled micro particles is examined. The shape, morphology, and size of the micro particles can be determined using laser light scattering and multi-size grating counter, in addition to experimental approaches [35,36].
Average particle size is determined by
D mean = Σ n d/Σ n
Where, n = number of micro particles checked; d = Mean size [24].
Angle of contact
The wetting property of the micro particle carrier is determined using the contact angle. Micro particles are classified according to their hydrophobicity or hydrophilicity. The existence of the adsorbed component controls this thermodynamic characteristic, which is specific to the solid. The contact angle is calculated at the solid/air/water interface. The advancing and receding contact angles are determined by placing the droplet in a circular cell over the objective of an inverted microscope. The contact angle is measured at 2000°C in one minute from when the micro particles are deposited [37].
Percentage yield
The entire amount of medication and polymer used in each batch is divided by the number of micro particles recovered from that batch, then divided by 100 [24].
Isoelectric point
The isoelectric point was calculated by micro electrophoresis, which was used to assess the electrophoretic mobility of the micro particles. The time it takes for particles to travel a distance of 1 mm at different PH values ranging from 3 to 10 is used to calculate average velocity. This data can be used to calculate a particle's electrical motion. The surface charge, ionizable behavior, and ion absorption features of micro particles can all affect their electrophoretic mobility [38].
Swelling index
It is calculated by finding out how many micro particles swell in a specific solvent. By soaking 5 mg of dry micro particles in 5 ml of buffer solution one night in to a measuring cylinder, the degree of equilibrium swelling of the micro particles can be calculated. It is determined using the formula provided [24].
Swelling index = mass of swollen micro particles minus mass of dry micro particles divided by mass of dry micro particles multiplied by 100.
Determining density
A multi-volume pycnometer can be used to determine the density of the micro particles. In the multi-volume pycnometer, a correctly weighted sample is put in a cup. The chamber is filled with helium and allowed to expand under continuous pressure. As a result of the expansion, the pressure inside the chamber lowers. The two consecutive pressure drop figures were obtained at different starting pressures. The density and volume of the micro particle carrier are determined using two pressure readings [39].
Fourier transforms infrared spectroscopy
The decadence of the carrier system's polymeric matrix is measured using Fourier transform IR spectroscopy. The surface of the micro particles is examined using ATR (alternate total reflectance). The infrared light that passed through the alternating total reflectance cell was reflected multiple times through to the sample, resulting in IR spectra that were dominated by surface materials. Alternating total reflectance FTIR spectroscopy can reveal the surface components of micro particles depending on manufacturing techniques and conditions [24].
Chemical analysis using electron spectrometry
ESCA [electron spectroscopy for chemical analysis] can be used to determine the surface chemistry of micro particles. In chemical analysis, electron spectroscopy can be used to identify the atomic structure of a surface. The surface degradation of biodegradable micro particles can be determined using spectra generated by electron spectrometry for chemical analysis [40].
Determining density
A multi-volume pycnometer can be used to determine the density of the micro particles. In the multi-volume pycnometer, a correctly weighted sample is put in a cup. The chamber is filled with helium and allowed to expand under continuous pressure. As a result of the expansion, the pressure inside the chamber lowers. The two consecutive pressure drop figures were obtained at different starting pressures. The density and volume of the micro particle carrier are determined using two pressure readings [39].
Fourier transforms infrared spectroscopy
The decadence of the carrier system's polymeric matrix is measured using Fourier transform IR spectroscopy. The surface of the micro particles is examined using ATR (alternate total reflectance). The infrared light that passed through the alternating total reflectance cell was reflected multiple times through to the sample, resulting in IR spectra that were dominated by surface materials. Alternating total reflectance FTIR spectroscopy can reveal the surface components of micro particles depending on manufacturing techniques and conditions [24].
Chemical analysis using electron spectrometry
ESCA [electron spectroscopy for chemical analysis] can be used to determine the surface chemistry of micro particles. In chemical analysis, electron spectroscopy can be used to identify the atomic structure of a surface. The surface degradation of biodegradable micro particles can be determined using spectra generated by electron spectrometry for chemical analysis [40].
Entrapment efficiency
A certain number of micro particles is weighed and crushed. After that, it was dissolved in buffer solution and filtered using a stirrer. A UV spectrophotometer is used to evaluate the filtrate at a given wavelength using a calibration curve [24].
The percentage of the experimental drug concentration to the theoretical drug concentration multiplied by 100 is the drug entrapment efficacy.
In vitro methods
This method can be used to determine the drug release profile and penetration of a medication through a barrier. The in vitro approach is used as a product testing technique in both pharmaceutical manufacture and product development. It's critical to have consistent and repeatable release data derived from chemically, physically and hydro dynamically established conditions [24].
Beaker method
In this operation, the dosage form is attached to the bottom of the medium beaker and is continuously agitated with an overhead stirrer. In literature research, the average volume employed ranges from 50 to 500 ml, with a mixing rate of 60 to 300 rpm [24].
Interface diffusion method
Dearden and Tomlinson came up with this approach. There are four different parts in all. Compartment A, which is first filled with a pharmaceutical concentration in buffer, represents the oral cavity. The buccal membrane is represented by compartment B, which has 1 octanol, while body fluids are represented by compartment C, which has 0.2 M HCl. Protein binding is represented by Compartment D, which also has 1-octanol. Before using; the watery phase and 1-octanol must be saturated. A syringe is used to retrieve samples from compartment A and return them to compartment A [24].
Modified Keshary Chien cell method
The use of advanced laboratory technology is required. At 37 degrees Celsius, it comprises of a Keshary Chien cell using distilled water (50 ml) as the dissolving medium. The TMDDS (Trans Membrane Drug Delivery System) is housed in a glass tube with a 10# sieve at the bottom that spreads with the help of the medium 30 times per minute [24].
Dissolution apparatus method
Using typical BP dissolving or USP apparatus, paddle and basket spinning elements have been employed to evaluate in vitro release properties. The study's dissolving media ranges from 100 to 500 ml, with rotation speeds of 50 to 100 rpm [24].
In vivo method
The transparency of intact mucous is determined using techniques that offer the biological response of the organism locally or systemically, as well as those that involve direct local examination of chemical uptake or aggregation on its surface. Animal models and buccal absorption investigations are two of the most commonly used in vivo research methods [24].
Animal models
It's mostly used to screen a large number of compounds, determine how they act, and evaluate a large number of formulations. Dogs, rats, pigs, and sheep are just a few of the animal models available. The technique includes anaesthetizing the animal, administering the dose, drawing blood at various intervals, and analyzing the results [24].
Buccal absorption test
For pharmaceutical combinations of one or several components, it is the most appropriate and reliable approach to determine the degree of loss of the drug from the human oral cavity. Adult subjects swish a 25 ml sample of the test solution for 15 min prior to expulsion to measure drug absorption kinetics while the medication is still in the mouth cavity. To find out how important the medicine is on a scale of importance structure, contact time, drug concentration at first use, and solution pH. The amount of drug remaining in the expelled volume is calculated to determine how much drug was absorbed [24].
Correlations between In vitro and In vivo
The association between in vitro dissolving rates and the rate and degree of availability as assessed by blood concentrations of the medication or metabolite and urinary excretion is referred to as ‘in vitro-in vivo correlations. Such links make it possible to create product criteria related to bioavailability [41,42].
IN VITRO DRUG DISSOLVED PERCENTAGE VS. PEAK PLASMA
Concentration
One the method to measure the association in vivo and in vitro is to calculate the percentage of drug released from various types of dosage forms, as well as the maximum plasma concentrations obtained by them, and compare them. Poorly made dosage forms are expected to release less drug than well-made dosage forms, resulting in a decreased amount of drug available for absorption [41,42].
Percentage of dissolved drug vs. percentage of absorbed drug
If the rate of dissolution is the limiting phase in drug absorption and the drug is totally absorbed after dissolution, a liner connection can be generated by comparing the percentage of drug absorbed with the percentage of drug dissolved. If the rate of absorption is the rate-limiting step in medication bioavailability, a change in dissolution rate may not be reflected in a change in the rate and extent of drug absorption from the dosage form [42,43].
Absorption rate vs. Dissolution rate
Generally, it is difficult to calculate the rate of absorption than it is to calculate the absorption time. Absorption time can be used to correlate absorption and dissolution data because the absorption rate and time of a drug are inversely connected. The absorption time of the dosage form can be used to differentiate between rapid and delayed absorption of the drug in the study of drug correlation in vitro and in vivo. The shorter the time it takes to absorb a specific amount of medicine, the faster it is absorbed. It has to do with how long it takes the same amount of medicine to be absorbed from the dose form [42,43].
Percentage of drug dissolved versus drug concentration in serum
For medications whose absorption from the gastrointestinal tract is limited by the rate of dissolution, a linear relationship can be constructed between the percentage of drug dissolved at particular periods and the serum drug concentrations at those times [42,43].
Percentage of dissolved drug vs. percentage of excreted dose in the urine
The percentage of a drug absorbed is proportional to the fraction of a drug dissolved. The weight of drug in the body and the weight of drug excreted in the urine have a link. As a result, a liner relationship between the amount of dissolved drug and the amount of released dose in the urine can be established [42,43].
RECENT ADVANCES IN TESTING OF MICRO PARTICLE
Advances in characterization of physicochemical properties of micro particles
Advancement in particle size morphological characterization
Laser light scattering/confocal fluorescence microscopy, light microscopy [LM], scanning electron microscopy [SEM] and multi-size grating counter are among the many methods used to assess particle size and the morphology of the micro particles. The most extensively utilized procedures for obtaining detailed information on the surface morphology of micro particles are optical LM and SEM. By seeing micro particles before and after the coating process, LM can be used to evaluate the micro particle coating parameter. Low resolution and the need for a high sample size to acquire trustworthy results are both disadvantages of LM.
SEM provides elemental information when paired with energy-dispersive or wavelength-dispersive X-ray spectroscopy. SEM is primarily used to investigate the form and surface of micro particles, as well as their cross sections, in order to reveal their internal structure. The images obtained by SEM have a higher resolution and are three-dimensional than those obtained by LM. Because electrons are used to build topographic and 3-D pictures, SEM has a higher resolution. The greatest resolution (distance between two items that can be separated and observed as different objects) of the SEM is 10-20 nm, but the LM's is 200-300 nm. If the material is not covered with a conductive substance, it will tend to charge in the electron beam, resulting in inaccurate scans and image abnormalities. As a result, SEM imaging sample must be covered with a very fine layer of an electrically conductive material like gold.
Micro particle sizes are often determined using light scattering methods. Simple sample preparation, no substantial experience required, quick measurement, and detailed results are all advantages of this procedure. However, massive particle interference, thick particle deposition, and multiple light scattering can all undermine the precision of the results. Because of the limits of individual procedures, it is sometimes necessary to employ a combination of techniques to determine particle size [44,45].
Advancement in Entrapment efficiency characterization
Entrapment efficiency refers to the capture efficiency of the drug, or the ratio of drug entrapment in the micro particles. A known amount of micro particles is dissolved in solvent (such as methanol and ethanol etc.) and free drug is released to determine drug content. A suitable solvent is utilized to dissolve or lyse the micro particles, depending on the solubility of the matrix and the active component. After that, the drug content is evaluated using an analytical technique such as high-performance liquid chromatography or according to the pharmacopoeial monograph. The following formula is used to compute the entrapment efficiency.
Actual content / Theoretical content x 100 Equals percent entrapment [44,46-48].
Advancement in Polymer molecular weight characterization
The first release pattern and duration of a micro particle matrix is influenced by the molecular weight of polymers. It is vital to remember that high shear processing (such as homogenization and ultrasonic mixing) as well as hydrolytic breakdown caused by a humid environment might influence polymer molecular weight [29]. Certain medications have the potential to expedite polymer decomposition, resulting in quicker release. As a result, keeping an eye on the molecular weight is crucial. Size exclusion chromatography is commonly used to find out the molecular weight of polymers (gel permeation chromatography) [49]. To ensure acceptable product performance (i.e., in vitro release) throughout the shelf life, a standard for an appropriate polymer molecular weight range should be established. The most commonly used polymer for micro particles, PLGA, is susceptible to deterioration from ionizing radiation, dampness, and high temperatures [29,44,50].
Advancement in Floating test characterization
This test is done to see if micro particles have the ability to float and, as a result, prolong GI retention. As defined by United States Pharmacopeia (USP) Device II, micro particles are dispersed throughout the surface of the release medium and permit to float or settle for a specified period of time under continuous stirring. At the last of the test time, the settled and floating micro particles are recovered one by one. Drying and weighing of the Micro particle Fractions the buoyancy of the micro particles is then estimated using the following equation.100 x (Qf/(Qf + Qs)) = buoyancy (percent)Qs And Qf are settled micro particle and the weight of the floating micro particles, respectively. The micro particles must float long enough for the drug to be completely released [33].
ADVANCEMENT IN MICRO PARTICLES
Characterization in In vivo buoyancy study
The in vivo transit behavior of buoyant and non-buoyant micro particle was assessed using scintigraphy in a rabbit model to establish that they provide appropriate stomach retention [2,51,-53].
Advance in the characterization of micro particles in the in vitro wash assay
The mucoadhesive characteristics of the micro particles are evaluated using an in vitro washout test. A piece of intestinal mucosa/mucous membrane is placed on a glass slide, a number of micro particles are spread on the tissue sample, and the slide is hung in a tablet disintegration machine. The disintegration machine is operated at low speed in an up and down motion using a suitable release medium (such as 0.1N HCl, pH 1.2 and phosphate buffer, pH 7.4). At various time periods, the number of micro particles still attached to the mucous membrane is counted [2,1152,53].
Advancement in swelling index characterization
The swelling index is commonly used for mucoadhesive micro particles. Before water ingress is established, the micro particles are suspended in a particular solvent and allowed to fully expand. After complete equilibrium, excess water sticking to the micro particles is removed using a soft tissue before weighing the swollen micro particles. After that, the micro particles are allowed to dry completely until no weight change is visible. The percentage of water uptake can then be calculated using the formula below.Weight of swollen micro particles - weight of dry micro particles / Weight of dry micro particles x 100 = percentage of water absorption [44].
Advancement in Drug-polymer interaction characterization
Interactions between the micro particle polymer and the encapsulated medication may result in poor delivery and possibly loss of therapeutic protein function. By comparing the drug’s IR spectra to the reference standard’s spectra, Fourier transform infrared [FT-IR] spectroscopy is frequently employed to discover any interactions between the medication and the polymer. The interaction between drug and polymer is indicated by changes in frequency and peaks. To measure polymer degradation, attenuated total reflectance FR-IR is employed to provide information on the surface composition of micro particles [11].
Advancement in Chemical analysis characterization
To analyze any surface degradation, electron spectroscopy is utilized to assess the atomic composition of micro particle surfaces. A brief description of this procedure is offered here because it is not commonly published. The photoelectric effect is used to emit electrons from the nucleus of the samples by means of an incident monochromatic X-ray beam. The top 10 nm of the micro particle surface's kinetic energy and number of electrons released are measured. The kinetic energies of these released electrons are equal to the X-ray energy minus the electrons' binding energy and the work function of the device. Binding energies specific to the particular elements may be determined from the kinetic energies of the released electrons, and the intensity of the binding energies can be utilized to quantify the particular element. This approach, on the other hand, has difficulty detecting hydrogen and helium. The detection limit is in the tens of thousands of parts per million ranges [44].
Advancement in Protein integrity determination characterization
During processing and in vitro release testing, encapsulated proteins are exposed to stress, which can lead to stability concerns (such as alteration of structural integrity, aggregation, denaturation, and loss of activity). Processing and/or release testing at a PH that corresponds to the protein's isoelectric point (pI) will cause considerable precipitation due to the molecule's low solubility at this PH. The determination of pI is done using a variety of electrophoretic techniques, including isoelectric focusing, gel electrophoresis, capillary electrophoresis, and pressure-mediated capillary electrophoresis. The pI value is used to ensure the structural integrity and stability of the encapsulated proteins. Polarization interferometry, nuclear magnetic resonance spectroscopy, X-ray crystallography can be used to identify protein structure and ensure its integrity [54-57].
Advancement in Density/porosity determination characterization
The density of the powder is used to determine flow and porosity. The most extensively used equipment for determining micro particle density is the multivolume pycnometer. Another approach for determining the porosity of micro particles is porosimetry, specifically mercury porosimetry [6].
Advancement in Contact angle characterization
Based on their wetting qualities, contact angle is used to describe the hydrophilicity/hydrophobicity of micro particles [6].
Advancement in Flow properties characterization
Because micro particles are powder dosage forms, determining flow parameters and avoiding segregation/dosage no uniformity is crucial. It's also important to understand the flow characteristics while packing and administering the completed drug. Measurement of flow characteristics is part of comparison testing and quality control. Tested flow parameters for micro particle products are tapped density, compressibility index, angle of response and true density. For example, the angle of repose of magnetic micro particles has been investigated.Flow properties are influenced by particle size and distribution, particle shape, chemical composition, moisture content, humidity, and temperature [58,59].
Advances in in vitro drug release testing
USP standard dissolution apparatus such as Apparatus IV [Flow-Through cell] and Apparatus II [rotating paddle] (flow-through cell) were used for the in vitro micro particle release test [60,61]. Non compendial techniques have also been used, such as simple and sort, dialysis bag and reverse dialysis bag. However, there are currently no standard in vitro release test procedures for micro particles. Various criteria (such as drug and polymer characteristics, micro particle properties, apparatus geometry and hydrodynamics, receiving media, and sink conditions) must be evaluated to develop the best in vitro release test procedures with a strong discriminatory capacity. The USP IV apparatus is currently the\ method of choice for micro particles. Other processes may be used, although regulatory authorities will normally require an explanation if the USP IV set is not used. In vitro release testing quality control procedures should be selective, reproducible and sensitive [43,62-65].
USP apparatus II (paddle type)
Micro particle release in vitro was studied using the USP apparatus II with and without dialysis sacs. When apparatus II is used without dialysis sacs, micro particles float on the surface of the release fluid, impeding medication release in some situations. When apparatus II was utilized alone rather than in conjunction with the dialysis sacs, the overall cumulative percentage release was found to be lower. Furthermore, USP Apparatus II mandates that the media be sampled and the micro particles be removed from the media for analysis, posing the risk of sample loss and mistake. In comparison to USP apparatus IV, the USP apparatus II takes longer and requires more people [66].
Sample and separate methods
Micro particle release investigations for research objectives frequently employ non-compendial sample and separate procedures. Micro particles are suspended in the release medium at a specific temperature (usually 37°C) and agitated continuously. Release samples are collected and centrifuged to separate the medium from the settled micro particles (if any). After each sample collection, an equivalent amount of fresh medium is supplied to the release medium container to keep the overall volume constant throughout the test. After certain periods of time, a complete replacement of the medium may be required to prevent drug degradation and/or maintain sink conditions. In sample and separate procedures, characteristics such as sample vial/vessel size, agitation speed, and sampling methods can be changed. Because of many limitations such as product aggregation and sample loss during the separation processes, sample and separate is not a reliable procedure, which might result in erroneous release profiles. Other drawbacks include centrifugal force disrupting the formulation and the use of vials/tubes/bottles of varying size, making inter-laboratory comparisons problematic [7,15,66].
Dialysis sac technique
The dialysis bag technique uses correct dialysis membranes with appropriate molecular weight cutoffs for the specific drug, passing the drug to simply flow with the help of the membrane into the release fluid, avoiding any type of interaction between the drug and the membrane [68]. The bag/dialysis bag is closed at both ends after introducing the micro particles (suspended in the release medium). The sack/dialysis bag is suspended in the appropriate release medium in the test container under constant agitation (accomplished using a shaker bath or paddle apparatus). To aid in drug dispersion, the volume of delivery medium in the bag is kept 5-10 times less than that in the test container.Test criteria to be evaluated include the rate of agitation, the volume of donor and acceptor cells, and the molecular weight of the dialysis membrane. The dialysis method has a number of advantages, including the ease with which samples can be extracted and the separation of micro particles. The dialysis bag method was successfully used with a peptide-loaded biodegradable micro particle system to obtain good correlation between in vivo and in vitro data, and in vitro data was used to determine micro particle performance in vivo [51]. The dialysis method has several disadvantages, including:
1. Obtaining enough agitation to obviate micro particle aggregation within the dialysis bags is problematic.
2. Insufficient agitation may cause a delay in reaching the equilibrium concentration of the drug.
3. Its use is restricted to drugs that do not bind to dialysis membranes.
4. Within the dialysis bag, there was a violation of the sink conditions.Sink conditions can be breached due to the little volume of release media inside the dialysis sacs and the dialysis sac membranes' small surface area. To address the issue of sink conditions being breached, the reverse dialysis process was developed. The released drug diffuses slowly into the dialysis sacs thanks to micro particles in the external release medium phase of this treatment [62].
USP apparatus IV method
The USP Pharmacologic IV Apparatus is now the preferred method for in vitro micro particle release testing. It can be done in both open and closed configurations, with different flow rates and temperatures, and with different types of flow-through cells [67]. Using a modified flow-through cell technique; micro particle drug release was investigated. In a modified flow-through method, the micro particles and glass beads are mixed. The glass beads are employed to:(1) Avoid micro particle aggregation and, as a result, a change in the percentage cumulative release due to changing surface area.
(2) Reduce dead volume within the cells.
(3) Improve laminar flow. To avoid backpressure, a proper ratio of glass beads to micro particles and appropriate filter types (used to filter the medium exiting the flow-through cells) must be utilized [44].The USP IV set simulates the injection site, and the constantly circulating medium around the micro particles mimics the dynamic environment in vivo, due to the limited volume of medium in flow-through cells (similar to subcutaneous tissues). Furthermore, compared to classical in vitro release protocols such as sample, strip and USP Apparatus II: No aggregation; data has the most cumulative release and the least volatility; sink conditions are easy to maintain. Multiple types of media (with varying pH and ionic strength) can be used; the flow rate can be controlled to control drug diffusion from the micro particles; and the medium can be easily changed with another medium type throughout the test if necessary. The USP IV device has several advantages over other methods when used in conjunction with fiber optic UV probes. The probes are withdrawn from the flow through cells and placed in medium reservoirs, reducing inaccuracy caused by suspended micro particles and air bubbles (due to agitation) interfering with the fiber-optic probes. The introduction of fiber optic probes enables for continuous monitoring of the first burst release of medication by the micro particles (where the release rate can be rapid) [69,70].The USP apparatus IV may be used to analyze both protein-loaded and small-molecule-loaded micro particles effectively. A comparison of the USP IV set with a different sample and technique was performed for the in vitro release test of protein-containing micro particles (bovine serum albumin). While protein adsorption on the hydrophobic surfaces of USP apparatus IV can cause an unexpected decrease in overall cumulative percent protein release, this can be avoided by using a suitable surfactant such as sodium dodecyl sulphate (SDS) [69,70].
The USP apparatus IV is made up of a number of important pieces, such as O-rings, filters, and valves, all of which must be in good working order for the apparatus to function properly. O-rings and filters may fail if apparatus IV is run for an extended period of time (weeks to months). Furthermore, tiny particle fragments, as well as polymer and polymer degradation products, can clog filters, causing backpressure issues. Modifications to the procedure, such as a change in the solvent and changes to the necessary portions, may be able to remedy these issues.
The modified USP apparatus IV method is regarded an excellent compendia dissolution method for micro particles due to its several advantages [69,70].
In vivo testing methods
Using various animal models, in vivo drug release testing is carried out to examine tissue distribution and pharmacokinetics of medicines released from micro particles. In vivo investigations are also carried out to assess drug and product stability. The following factors should be considered while designing in vivo release tests:
- Choosing an appropriate animal model that takes into account the animal's lifespan, especially when testing formulations with a long duration of action.
- Antibodies could be produced while employing human-derived protein therapies due to immunogenicity, which could influence medication pharmacokinetics and pharmacodynamics of proteins [33,71].
Following literature research and a comparison of the injection site in animals and humans to evaluate any inter-species variances, an appropriate animal model can be chosen. For micro particle performance testing, animal models such as rats and rabbits have been routinely used [35].
Blood or urine samples are collected over a period of time after micro particles are delivered. Different extraction processes (such liquid-liquid and protein precipitation) are employed to extract drugs from the biological matrix. To assess the extracted chemicals, researchers use a variety of techniques, including liquid chromatography-mass spectrometry, ultra-performance liquid chromatography-tandem mass spectrometry and liquid chromatography-tandem mass spectroscopy. In vivo drug release from micro particles is influenced by two types of variables: factors that are dependent on the delivery system and factors that are not dependent on the delivery system (such as increased drug release as result of enzymatic deterioration of the polymers and phagocytosis; such as reduced drug release as a result of protein adsorption) characteristics and considerations unrelated to delivery system (such as food, fluid viscosity, and connective tissue that limit drug diffusion, drug absorption into fatty tissues that affect drug partitioning, as well as fluid volume and muscle movement at the injection site that affect the volume available for drug dissolution and systemic absorption). In addition, fasting and fed conditions alter drug release and bioavailability after oral administration of micro particles. Micro particles spend more time in the stomach when they feed, delaying the amount of drug accessible at the site of action [72].
RECENT ADVANCEMENT OF CHITOSAN POLYMER
Effects on cholesterol levels
As examples of fibers with high, intermediate and low bile acid binding capabilities, chitosan and cellulose were chosen. Liquid cholesterol levels nearly doubled to 4.3mm in a control group of mice fed a high fat/high cholesterol diet for three weeks, but inclusion of either of these fibers in 7.5 percent of the diet prevented this increase furthermore, when these fibers were provided, the HFHC diet lowered the quantity of cholesterol deposited in liver storage. Although all three fiber types were hypercholesterolemia, cholestyramine induced the greatest loss of cholesterol in liver tissue. Reduce cholesterol intake (food) decreased cholesterol absorption efficiency and increased fecal excretion of bile acids and cholesterol where the processes behind cholestyramine’s strong bile acid binding ability are responsible for the latter effects. On the other hand, cellulose or chitosan lowered cholesterol absorption or fecal sterol production [73,74].
Increase Stability of Drug
Combining a drug with chitosan, generating a suspension and then kneading it for 45 minutes until a dough develops the stability of the medicine. This dough mass is screened 16 times to produce granules that are entirely stable in all situations [74].
Patients with orthopedic problems
Chitosan is a biopolymer with healing and antimicrobial properties, making it an excellent material for bioactive coatings for orthopedic. It has been proven to promote wound healing, bone regeneration and tissue growth [75].
Cosmetic Industry
The presence of unique quaternary chitosan derivatives in the formula distinguishes cosmetic formulations for hair or skin treatment. Chitosan derivatives have a high molecular weight and have been demonstrated to strengthen and condition hair when compared to hair keratin [75,76].
As dental drug
Chitosan has been demonstrated to hasten wound recovery and protect the formation of excessive scars, resulting in a more attractive skin surface. Chitosan is also used as a dressing for oral mucosal lesions and as a buffer following intensive maxillary sinusitis therapy. It is also being investigated as an absorbent membrane for periodontal surgery. Chitosan has a broad range of biological activities and is advertised as a health food that can help treat a broad range of ailments, including diabetes, hepatitis, arthritis, cancer and more [76].
Chitosan as Permeation Enhancer
Chitosan has been proven to unclosed inflexible junctions in cell membranes due to its cationic characteristics. This feature has prompted interest in using chitosan as a penetration enhancer for hydrophilic medicines like peptides that have poor oral bioavailability. Because interactions between the positive charges on the and the cell membrane produce absorption amplification, the phenomena is concentration and pH dependent. Increased permeability would be obtained by raising the polymer's charge density [74,76].
As a Mucoadhesive Excipient, Chitosan
By increasing the duration, a medicine spends in the intestinal tract; bio adhesiveness is commonly utilized to boost oral bioavailability. When chitosan is compared to other commonly used polymeric excipients including cellulose, Xanthan gum, and starch, the cationic polymer outperforms the natural polymers in terms of bio adhesion [74] (Table 1).
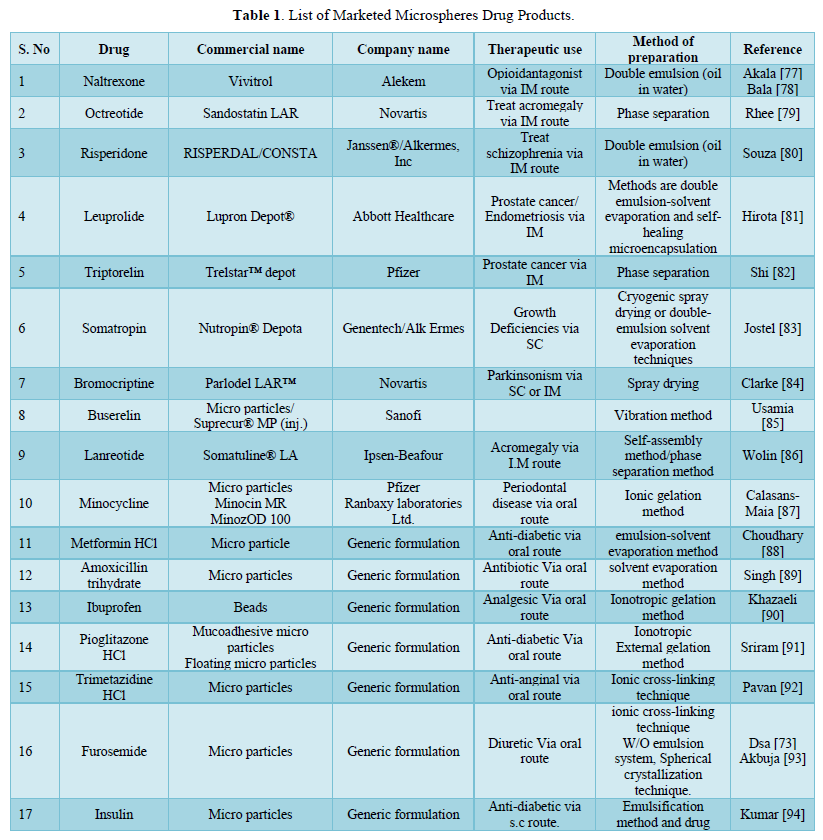
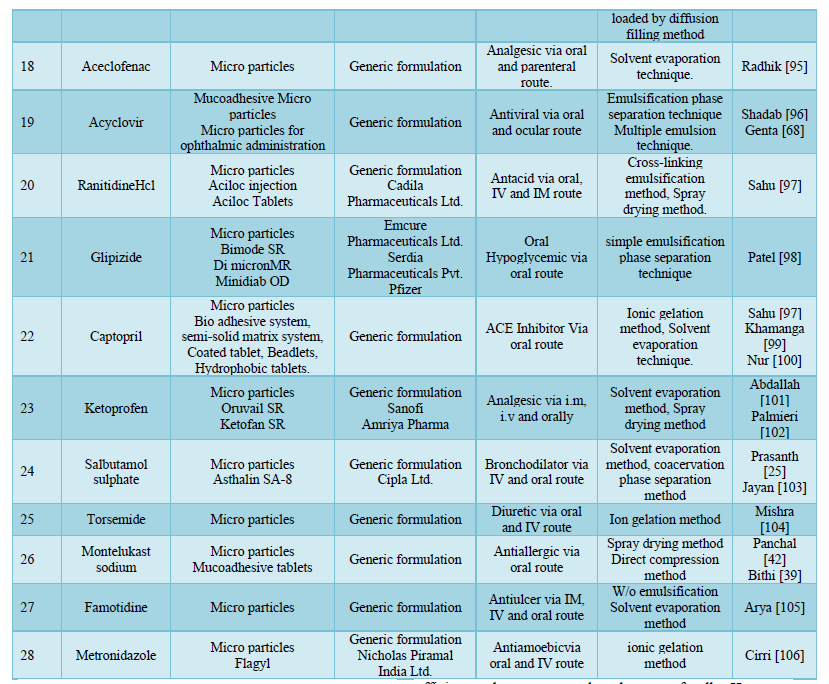
MICRO PARTICLE APPLICATIONS
Delivery of vaccines with micro particles
A vaccination must defend against the bacteria or its harmful product. These features should be included in an ideal vaccine: safety, ease to use, efficacy, and affordability. It's challenging to strike a balance between safety and minimizing unpleasant reactions. The degree, to which the antibody response is formed, as well as the query of safety, is both intimately tied to the application strategy. Traditional vaccines have flawed those biodegradable vaccine delivery systems for parenteral immunizations can remedy [40].
Gene delivery using micro particles
Viral vectors, non-ionic liposomes, polycationic complexes and microcapsules are used to deliver genetic drugs. Virus vectors are ideal for genotype delivery because they are very efficient and can target a broad range of cells. However, when administered in vivo, they cause immunological reactions and have deleterious consequences. Non-viral delivery techniques for gene therapy have been studied to overcome the limitations of viral vectors. Non-viral delivery approaches provide a number of merits, including ease of preparation, cell/tissue targeting, decreased immune response, unrestricted plasmid size, and repeatable large-scale output. The polymer will be used in gene delivery applications as a DNA carrier [107-109].
Using micro particle carriers to target
Pharmaceutical site-specific distribution, also known as targeted medication delivery, is a well-known concept that is getting a lot of traction. A drug's therapeutic efficacy is determined by its capability to reach and engage target receptors. Drug activity mediated by the utilization of a transporter system is linked to the ability to efficiently, consistently and specifically exit the group [22].
Monoclonal antibodies facilitated micro particles targeting
Monoclonal antibodies that attack micro particles are known as immune micro particles. This approach is used to precisely target specific websites. Monoclonal antibodies are molecules with an extremely limited range of applicability. Micro particles containing bioactive substances can be targeted to specific sites using monoclonal antibodies (Mabs) with high specificity. Mabs can directly bind to micro particles thanks to covalent coupling. The antibodies can bind to amino, free aldehyde or hydroxyl groups on the shell of the micro particles. Attaching maps to micro particles can be done in a number of ways;
- Adsorption, both nonspecific and selective
- Direct coupling
- Reagent coupling [22].
Chemotherapy with micro particles
Micro particles as carriers of anticancer medicines are one of the most promising applications. Micro particles were required due to increased leaky vasculature and endocytic activity. Covering the soluble polyoxymethylenemicro particles creates invisible micro particles. Non-stealth micro particles that accumulate in the RES [Reticulo Endothelial System] could also be used to treat cancer [16].
Imaging
Micro particles have been thoroughly researched and used in a variety of applications. Radioactively labeled micro particles can be used to imaging tissues, organs, cell lines and various cells. When imaging specific regions, the particle size range of micro particles becomes a serious concern. Particles inserted into a vein other than the portal vein become stuck in the lungs' capillary bed. Using tagged human serum albumin micro particles; this phenomenon is employed to get scintigraphy images of tumor masses in the lungs [24].
Micro particles with porous surfaces that can be applied to the skin
Micro sponges are porous micro particles with several interconnected voids that range in size from 5 to 300 microns. These porous micro particles with active compounds can be used in creams, lotions, and powders, and can trap different types of active components such as fragrances, essential oil sand emollients. Micro sponges are non-foldable structures with a porous surface that slowly release active chemicals [24].
Nasal medication administration
Intranasal (IN) administration provides a number of practical and theoretical merits for systemic and local delivery of a variety of therapeutic substances. Intranasal delivery is painless, doesn't require needles, and doesn't necessitate sterile preparation. It's also self-contained. A great number of microvilli, a penetrable endothelium membrane, and a highly vascularized epithelium in the nasal mucosa contribute to fast beginning of therapeutic impact. It covers a wide range of drug administration methods, equipment, formulations, and procedures for the nose and nasal cavity. Intranasal drugs can be used for local or systemic treatment, depending on the therapeutic goal. It is critical to couple the bio adhesive properties to the micro particles due to the additional benefits of efficient drug absorption and increased bioavailability, much closer contact with the mucosal layer and a reduction in the frequency of drug administration due to reduced mucociliary clearance of adhering drug delivery systems to the nasal mucosa by administering medication through the nose [24].
Controlled gastro protective delivery system
Buoyant systems are low-density systems that float on gastric contents and remain in the stomach for longer periods of time than usual dosage forms. The ability to adjust the emptying time of dosage forms is a great advantage for dosage forms, since stomach emptying is such a fickle process. Building controlled release systems to improve absorption and bioavailability, on the other hand, presents a number of obstacles. The medicine is administered slowly and at the correct rate since the system floats above the gastric contents, resulting in less variation in plasma drug concentration and longer gastronomy retention time. Polymers such as polyvinyl acetate, Eudragit, Methocil, agar, polycarbonates, cellulose acetate, chitosan and other polymers are utilized in gastro protective controlled release systems [24].
Implantable gadgets
In the medical field, microencapsulation has been used to encapsulate live cells. Encapsulating artificial cells and macromolecules including hormones, peptides and proteins improves biocompatibility, preventing undesired immunological reactions that could result in rejection or inactivation. The micro particles are used to keep the components separate until they are required to function. Micro particles are employed in the biotechnology industry to help separate organisms and their recombinant products [24].
Oral medication administration
Rabbits were used to investigate the possibility of a polymer matrix carrying diazepam as an oral medication delivery mechanism. He discovered that a film constructed from a 1:0.5 ratio of medication and polymer could have been a viable alternative to typical tablet formulations. Polymers' capacity to form films could lead to the creation of film dosage forms as a replacement for medicinal pills. When paired with the amine group's two major processes, the pH sensitivity of the polymer begins to distinguish it as a one-of-a-kind polymer for oral drug administration applications [24].
Ocular delivery micro particles
Glaucoma is treated with the majority of drug-loaded ocular delivery devices, especially cholinergic agonists such as pilocarpine. Micro particles having biodegradable properties from a very short period [1 to 3 min] can be used to extend the low elimination half-life of aqueous eye drops to a larger length [15-20 min]. For instance, polyalkylcyanoacrylate [37].
APPLICATIONS FOR PHARMACEUTICALS
Microencapsulated pharmaceuticals presently in the market include progesterone, theophylline, aspirin and derivatives, antihypertensive, pancrelipase, potassium chloride. Microencapsulated Potassium Chloride is used to protect intestinal problems that may be caused by potassium chloride. The microcapsules' dispersibility and the ions' regulated release reduce the risk of excessive localized salt concentrations, which can lead to perforation, ulceration and bleeding. Injectable and inhalation treatments containing micro particles have also been proposed. The amounts of research done in this subject or the benefits that can be realized with this technology are not reflected in the number of commercially accessible items. Cost factors have influenced the quantity of medicinal microencapsulated goods. The majority of encapsulation procedures is costly and necessitates a substantial money investment in appliance. Spray or drum coating and spray drying are exceptions, as the requisite appliance may already be on hand within the company. The fact that most microencapsulation techniques are patent protected adds to the cost [107].
CONCLUSION
The study summarizes key physicochemical aspects of microspheres, such as drug content, particle size and thermal characteristics, as well as advances in characterization approaches. Micro particles in vitro release testing is a significant physicochemical attribute. Micro particles are tested using a variety of compendial and non-compendial in vitro release-testing procedures, including USP equipment IV, dialysis membrane sacs and sample and separate. Based on a comparison of the respective benefits and drawbacks of various methods, modified USP apparatus IV appears to be the method of choice at the moment. Quality control measures such as high temperature have been used to design accelerated in vitro release tests. These accelerated release-testing techniques must be highly correlated with in vitro real-time release-testing procedures. To reduce the necessity for in vivo performance evaluation, IVIVCS based on real-time and accelerated release data can be produced. To investigate the impact of wide range of environmental conditions on microsphere quality over the product's life span, storage stability studies are required. Various novel assays have been created, including the In vitro wash off test and the floating test, as well as improvements in physicochemical feature characterization approaches. The micro particles drug delivery system is the most preferred medication administration system due to its benefits of controlled and sustained release action, better stability, lower frequency of administration, dissolving rate and bioavailability. The micro particles are spherical microspheres that are used to deliver the medicine to the target location with pinpoint accuracy if customized, and to maintain the optimum concentration at the place of interest without side effects. The medicine is contained within a unique polymeric membrane in the center of the micro particles. Micro particles will play a very important and central role in novel drug delivery in the upcoming years, particularly in diseased cell sorting, diagnostic test, targeted, secure, specific & effective in vitro delivery & supplements as smaller versions of damaged tissue & organs in the body, by combining different other techniques.
- Nikam VK, Gudsoorkar VR, Hiremath SN, Dolas RT, Kashid VA (2012) Microspheres-A Novel drug delivery system: An overview. Int J Pharm Chem 1(1): 113-128.
- Rastogi V, Shukla SS, Singh R, Niharika L, Yadav P (2016) Micro particles: A Promising Drug Carrier. J Drug Deliv Ther 6(3): 18-26.
- Chaudhari A, Jadhav RK, Kadam JV (2010) An overview: Microspheres as a nasal drug delivery system. Int J Pharm Sci Rev Res 5(1): 8-17.
- Tarun Virmani, Gupta J (2017) Pharmaceutical Application of Microspheres: An Approach for the Treatment of Various Diseases. Int J Pharm Sci Rev Res 8(8): 3252-3260.
- Prasad BSG, Gupta VRM, Devanna N, Jayasurya K (2014) Microspheres as Drug Delivery System - A Review, J Glob Trends Pharm Sci 5(3): 1961-1972.
- Alagusundaram M, Madhu SCC, Umashankari K, Attuluri VB, Lavanya C, et al. (2009) Microspheres as a novel drug delivery system - a review. Int J ChemTech Res 1: 526-534.
- Lehr C-M, Bouwstra JA, Tukker JJ, Junginger HE (1990) Intestinal transit of bio adhesive microspheres in an in-situ loop in the rat - a comparative study with copolymers and blends based on poly (acrylic acid). J Control Release 13: 51-62.
- Okubo M, Kondo Y, Takahashi M (1993) Production of submicron-size monodisperse polymer particles having aldehyde groups by seeded aldol condensation polymerization. Colloid Polym Sci 271: 109-113.
- Kojima T, Nakano M, Juni K, Inoue S, Yoshida Y (1984) Preparation and Evaluation in vitro of Polycarbonate Microspheres Containing Local Anesthetics. Chem Pharm Bull 32(7): 2795-2802.
- Mahale MM, Saudagar RB (2019) Microsphere: A review. J Drug Deliv Ther 9(3): 854-856.
- Kumar A, Mahajan S, Bhandari N (2017) Microspheres: A review. World J Pharm Pharm Sci 6: 724-740.
- Meghna KS, Krishna MP, Giridas S, Sreelakshmi C, Vijayakumar B (2017) Microsphere a drug delivery system-A review. Int J Novel Trends Pharm Sci 7: 109-118.
- Khamanga SM, Walker RB (2021) In vitro dissolution kinetics of captopril from micro particles manufactured by solvent evaporation. Dissolution Technol 19(1): 42-52.
- Dutta P, Sruti J, Patra CN, Rao M (2011) Floating microspheres: Recent trends in the development of gastro retentive floating drug delivery system. Int J Pharm Sci Nanotech 4(1): 1296-1206.
- Hafeli V (2002) Radioactive microsphere for medical application. Adv Chem Phys 7: 213-248.
- Vyas SP, Khar RK (2004) Targeted and Controlled Drug Delivery, Novel Carrier Systems, first reprint. pp: 418-454.
- Desai S, Bolton S (1993) A floating controlled release drug delivery system: In-vitro - in-vivo Pharm Res 10: 1321-1325.
- Iannaccelli V, Coppi G, Bernabei MT, Cameroni R (1998) Air compartment multiple unit system for prolonged gastric residence: Part 1. Formulation study. Int J Pharm 174: 47-54.
- Najmuddin M, Ahmed A, Shelar S, Patel V, Khan T (2010) Floating microspheres of ketoprofen: Formulation and evaluation. Int J Pharm Pharm Sci 2(2): 83-87.
- Trivedi P, Verma AML, Garud N (2008) Preparation and Characterization of Acclofenac Microspheres. Asian J Pharm 2(2): 110-115.
- Saralidze K, Koole LH, Knetsch MLW (2010) Polymeric Microspheres for Medical Applications. Materials 3: 3357-3364.
- Nair R, Reddy B (2009) Application of chitosan microspheres as drug carriers: A review. J Pharm Sci Res 1: 1-12.
- Orienti I, Aiedeh K, Gianasi E, Bertasi V, Zecchi V (1996) Indomethacin loaded chitosan microspheres correlation between the erosion process and release kinetics. J Microencapsul 13: 463-472.
- Das MK, Ahmed AB, Saha D (2019) Microsphere a drug delivery system: A review. Int J Curr Pharma Res 11: 34-41.
- Prasanth VV, Moy AC, Mathew ST, Mathapan R (2011) Microspheres An overview. Int J Res Pharm Biomed Sci 2: 332-338.
- Prasanth VV, Chakraborty A, Mathew ST, Mathappan R, Kamalakkannan V (2011) Formulation and evaluation of Salbutamol sulphate micro particles by solvent evaporation method. J Appl Pharm Sci 1(5): 133-137.
- Kumar R, Palmieri MJ Jr (2010) Points to consider when establishing drug product specifications for parenteral microspheres. AAPS J 12: 27-32.
- Kawashima Y, Niwa T, Takeuchi H, Hino T, Ito Y (1991) Preparation of multiple unit hollow microspheres (microballoons) with acrylic resin containing tranilast and their drug release characteristics (in vitro) and floating behavior (in vivo). J Control Release 16: 279-289.
- Kawashima Y, Niwa T, Takeuchi H, Hino T, Ito Y (1991) Preparation of multiple unit hollow microspheres (microbal loons) with acrylic resin containing tranilast and their drug release characteristics (in vitro) and floating behavior (in vivo). J Control Release 16: 279-290.
- Suvarna V, Kadam NV (2015) Microspheres: A brief review. Asian J Biomed Pharm Sci 5(47): 13-19.
- Hickey T, Kreutzer D, Burgess DJ, Moussy F (2002) In vivo evaluation of a dexamethasone/PLGA microsphere system designed to suppress the inflammatory tissue response to implantable medical devices. J Biomed Mater Res 61: 180-187.
- Yadav R, Bhowmick M, Rathi V, Rathi J (2019) Design and characterization of floating microspheres for rheumatoid arthritis. J Drug Deliv Ther 9(2-s): 76-81.
- Barkai A, Pathak V, Benita S (1990) Polyacrylate (Eudrugit retard) microspheres for oral controlled release of nifedipine. I. Formulation design and process optimization. Drug Dev Ind Pharm 16: 2057-2075.
- Jain SK, Agrawal GP, Jain NK (2006) A novel calcium silicate-based microspheres of repaglinide: In vivo J Control Release 113: 111-116.
- Patil NV, Wadd NV, Thorat SS, Upadhye SS (2020) Microspheres: A novel drug delivery system. Am J Pharm Tech Res 10(2): 286-301.
- Sinha VR, Agrawal MK, Kumria R (2005) Influence of formulation and excipient variables on the pellet properties prepared by extrusion spheronization. Curr Drug Deliv 2: 1-8.
- Bithi FA, Saha T, Ahmed N, Hasan I, Md Selim R (2017) Preparation and in vitro evaluation of mucoadhesive tablets of montelukast sodium. Bangladesh Pharm J 20(2): 123-131.
- Kawashia Y, Niwa T, Takeuchi H, Hino T, Itoh Y, et al. (1991) Characterization of polymorphs of tranilast anhydrate and tranilast monohydrate when crystallized by two solvent change spherical crystallization techniques. J Pharm Sci 81: 472-478.
- Bodmeier R, Chen H (1989) Preparation and characterization of microspheres containing the anti-inflammatory agents, Indomethacin, ibuprofen and kitoprofen. J Control Release 10: 167-175.
- Panchal R, Patel H, Patel V, Joshi P, Parikh A (2012) Formulation and evaluation of montelukast sodium - chitosan based spray dried microspheres for pulmonary drug delivery. J Pharm Bioallied Sci 4(Suppl 1): S110-S111.
- Brahmankar DM, Sunil BJ (2005) Biopharmaceutics and Pharmackinetics- A Treatise. Vallabh Prakashan, Delhi. pp: 315-336.
- Andhariya JV, Burgess DJ (2016) Recent advances in testing of micro particle drug delivery systems. Expert Opin Drug Deliv 13(4): 1-16.
- Prajapati SK, Tripathi P, Ubaidulla U, Anand V (2008) Design and development of gliclazide mucoadhesive microcapsules: In vitro and in vivo AAPS Pharm Sci Tech 9: 224-230.
- Jain NK (2004) Controlled and Novel drug delivery, CBS Publishers New Delhi, India, 4th pp: 236-237,21.
- Jain SK, Agrawal GP, Jain NK (2006) Evaluation of porous carrier- based floating orlistat microspheres for gastric delivery. AAPS Pharm Sci Tech 7: E54-E62.
- Mukund J, Bhujbal R, Ranpise N (2012) Floating microspheres: A review. Braz J Pharm Sci 48: 17-30.
- Murty SB, Goodman J, Thanoo BC, Deluca PP (2003) Identification of chemically modified peptide from poly (D, L-lactide-coglycolide) microspheres under in vitro release conditions. AAPS Pharm Sci Tech 4: 392-405.
- Kadajji GV, Betageri GV, Venkatesan N (2011) Approaches for dissolution testing of novel drug delivery systems. Am Pharm Rev 14(6): 38-44.
- Kostanski JW, DeLuca P (2000) A novel in vitro release technique for peptide-containing biodegradable microspheres. AAPS Pharm Sci Tech 1(1): 30-40.
- Rathinaraj BS, Rajveer CH, Sudharshini S, Reddy KA (2010) Preparation and evaluation of mucoadhesive microcapsules of Nimodipne. Int J Res Pharm 1: 219-224.
- Reddy VM, Patil P, Biradar KV, Dana SB, Kumar DN (2011) Development and evaluation of mucoadhesive microspheres of Nimodipine. Int Res J Pharm 2: 91-98.
- Glukhovskiy PV, Vigh G (1998) A simple method for the determination of isoelectric points of ampholytes with closely spaced pKa values using pressure-mediated capillary electrophoresis. Electrophoresis 19: 3166-3170.
- Park TG (1994) Degradation of poly (D, L-lactic acid) microspheres: Effect of molecular weight. J Control Release 30: 161-173.
- Righetti PG, Gelfi C, Perego M, Stoyanov AV, Bossi A (1997) Capillary zone electrophoresis of oligonucleotides and peptides in isoelectric buffers: theory and methodology. Electrophoresis 18: 2145-2153.
- Varcheh NN, Luginbuehl V, Aboofazeli R, Merkle HP (2011) Preparing poly (lactic-co-glycolic acid) (PLGA) microspheres containing lysozyme-zinc precipitate using a modified double emulsion method. Iran J Pharm Res 10: 203.
- Chandna A, Batra D, Kakar S, Singh R (2013) A review on target drug delivery: Magnetic microspheres. J Acute Dis 2: 189-195.
- Kawatra M, Jain U, Ramana J (2012) Recent advances in floating microspheres as gastro-retentive drug delivery system: A review. Int J Recent Adv Pharm Res 2: 5-23.
- Aklonis JJ, MacKnight WJ (1983) Transitions and relaxations in amorphous polymers. In: Aklonis JJ, MacKnight WJ, editors. Introduction to polymer viscoelasticity. 2nd New York: Wiley-Interscience.
- Shen J, Burgess DJ (2012) Accelerated in vitro release testing methods for extended-release parenteral dosage forms. J Pharm Pharmacol 64: 986-996.
- Chidambaram N, Burgess DJ (1999) A novel in vitro release method for submicron sized dispersed systems. AAPS Pharm Sci Tech 1: E11.
- Kokkona M, Kallinteri P, Fatouros D, Antimisiaris SG (2000) Stability of SUV liposomes in the presence of cholate salts and pancreatic lipases: Effect of lipid composition. Eur J Pharm Sci 9: 245-252.
- Vemuri S, Yu C, Pushpala S (1991) Drug release rate method for a liposome preparation. Drug Dev Ind Pharm 17: 183-192.
- Xiao C, Qi X, Maitani Y, Nagai T (2004) Sustained release of cisplatin from multivesicular liposomes: Potentiation of antitumor efficacy against S180 murine carcinoma. J Pharm Sci 93: 1718-1724.
- Wei Y, Zhao L (2008) In vitro and in vivo evaluation of ranitidine hydrochloride loaded hollow microspheres in rabbits. Arch Pharm Res 31: 1369-1377.
- Burgess DJ, Crommelin DJA, Hussain AJ, Chen M-J (2004) EUFEPS workshop report, assuring quality and performance of sustained and controlled release parenterals. Eur J Pharm Sci 21: 679-690.
- Genta I, Conti B, Perugini P, Pavanetto F, Spadaro A, et al. (1997) Bio adhesive micro particles for ophthalmic administration of acyclovir. J Pharm Pharmacol 49(8): 737-742.
- Voisine J, Zolnik B, Burgess DJ (2008) In situ fiber optic method for long-term in vitro release testing of microspheres. Int J Pharm 356: 206-211.
- Zolnik BS, Raton J-L, Burgess DJ (2005) Application of USP apparatus 4 and in situ fiber optic analysis to microsphere release testing. Dissolution Technol 12: 11-14.
- Joseph N, Lakshmi S, Jayakrishnan A (2002) A floating- type oral dosage form for piroxicam based on hollow polycarbonate microspheres: In vitro and in vivo evaluation in rabbits. J Control Release 79: 71-79.
- Sato Y, Kawashima Y, Takeuchi H, Yamamoto H, Fujibayashi Y (2004) Pharmacoscintigraphic evaluation of riboflavin-containing micro balloons for a floating controlled drug delivery system in healthy humans. J Control Release 98: 75-85.
- Das MK and Senapati PC (2007) Evaluation of furosemide-loaded alginate microspheres prepared by ionotropic external gelation technique. Acta Pol Pharm 64(3): 253-262.
- Yong Z, Lili C, Feng L, Nianqiu S, Chunlei L, et al. (2016) Design, fabrication and biomedical applications of zein-based nano/micro-carrier systems. Int J Pharm 512: 191-210.
- Khandai M, Chakraborty S, Sharma A, Pattnaik S, Patra ChN, et al. (2010) Preparation and evaluation of algino-sericin mucoadhesive microspheres: An approach for sustained drug delivery. J Adv Pharm Res 1: 48-60.
- Virmani T and Gupta J (2017) Pharmaceutical application of microspheres: An approach for the treatment of various diseases. Int J Pharm Sci Res 8(8): 3252-3260.
- Akala EO, Wiriyacoonkasem P, Pan G (2011) Studies on in vitro availability, degradation, and thermal properties of naltrexone-loaded biodegradable micro particles. Drug Dev Ind Pharm 37(6): 673-684.
- Bala M, Moudgil A, Kumar S (2020) An Update on Biodegradable Micro particles Loaded with Naltrexone. Int J Pharma Res Health Sci 8(2): 3143-3146.
- Rhee Y-S, Sohn M, Woo BH, Thanoo BC, DeLuca PP, et al. (2011) Sustained-release delivery of octreotide from biodegradable polymeric micro particles. AAPS Pharm Sci Tech 12(4): 1293-1301.
- Souza S, Faraj JA, Giovagnoli S, Deluca PP (2014) Development of risperidone PLGA micro particles. J Drug Deliv 2014: 1-11.
- Hirota K, Doty AV, Ackermann R, Zhou J, Olsen KF, et al. (2016) Characterizing release mechanisms of leuprolide acetate-loaded PLGA micro particles for IVIVC development I: In vitro J Control Release 244: 302-313.
- Shi Y, Li LC (2005) Current advances in sustained-release systems for parenteral drug delivery. Expert Opin Drug Deliv 2(6): 1039-1058.
- Jostel A, Shalet SM (2006) Prospects for the development of long-acting formulations of human somatropin. Treat Endocrinol 5(3): 139-145.
- Clarke N, O'Connor K, Ramtoola Z (1998) Influence of Formulation Variables on the Morphology of Biodegradable Micropartieles Prepared by Spray Drying. Drug Dev Ind Pharm 24(2): 169-174.
- Usami M, Misawa K, Yagic N, Sekikawac H, Nabeshima T (2007) Buserelin acetate micro particle dispersion affects drug release and plasma E1 levels. Int J Pharm 339(1-2): 130-138.
- Wolin EM, Manon A, Chassaing C, Lewis A, Bertocchi L, et al. (2016) Lanreotide depot: An antineoplastic treatment of carcinoid or neuroendocrine tumors. J Gastrointest Cancer 47(4): 366-374.
- Calasans-Maia MD, Barboza Junior CAB, Soriano-Souza CA, Alves ATNN, Uzeda MJdeP, et al. (2019) Micro particles of alginate encapsulated minocycline-loaded nanocrystalline carbonated hydroxyapatite: Therapeutic potential and effects on bone regeneration. Int J Nanomedicine 14: 4559.
- Choudhury PK, Kar M (2009) Controlled release metformin hydrochloride micro particles of ethyl cellulose prepared by different methods and study on the polymer affected parameters. J Microencapsul 26(1): 46-53.
- Singh SK, Chidrawar VR, Ushir YV, Vadalia KR, Sheth NR, et al. (2010) Pharmaceutical characterization of amoxicillin trihydrate as mucoadhesive micro particles in management of pylori. Int J PharmTech Res 2(1): 348-358.
- Khazaeli P, Pardakhti A, Fereshteh H (2008) Formulation of ibuprofen beads by ionotropic gelation. Iran J Pharm Res 7(3): 163-170.
- Sriram N, Katakam P (2016) Formulation and evaluation of mucoadhesive micro particles of pioglitazone hydrochloride prepared by ionotropic external gelation technique. J Encapsulation Adsorpt Sci 6(1): 22-34.
- Pavanveena C, Kavitha K, Kumar SNA, Mani TT (2010) Formulation and evaluation of trimetazidine hydrochloride loaded chitosan micro particles. Int J Pharm Pharm Sci 2: 67-70.
- Akbuja J, Durmaz G (1994) Preparation and evaluation of cross-linked chitosan micro particles containing furosemide. Int J Pharm 111(3): 217-222.
- Kumar TM, Paul W, Sharma CP (2005) Bioadhesive, pH responsive micromatrix for oral delivery of insulin. Trends Biomater Artif Organs 18(2): 198-202.
- Radhika P, Luqman M, Borkhataria C (2008) Preparation and evaluation of delayed release aceclofenac micro particles. Asian J Pharm 2(4): 252-254.
- Shadab Md, Ahuja A, Khar RK, Baboota S, Chuttani K, et al. (2011) Gastroretentive drug delivery system of acyclovir-loaded alginate mucoadhesive micro particles: Formulation and evaluation. Drug Deliv 18(4): 255-264.
- Sahu S, Chourasia A, Toppo A, Asati A (2012) Formulation and evaluation of captropril micro particles by ionic gelation technique. Int J Pharm Sci 3: 1377-1379.
- Patel JK, Patel RP, Amin AF, Patel MM (2005) Formulation and evaluation of mucoadhesive glipizide micro particles. AAPS PharmSciTech 6(1): E49-E55.
- Nur AO, Zhang JS (2000) Recent progress in sustained/controlled oral delivery of captopril: An overview. Int J Pharm 194(2): 139-146.
- Abdallah MH, Sammour AO, El-ghamry HA, Elnahas HM (2012) Development and characterization of controlled release ketoprofen micro particles. J Appl Pharm Sci 2(3): 60-67.
- Palmieri GF, Bonacucina G, Di Martino P, Martelli S (2002) Gastro-resistant micro particles containing ketoprofen. J Microencapsul 19(1): 111-119.
- Jayan SC, Sandeep A, Rifash M, Mareema CM, Shamseera S (2009) Design and in-vitro evaluation of gelatin micro particles of salbutamol sulphate. Hygeia 1(1): 17-20.
- Mishra B, Sahoo S, Biswal PK, Sahu SK (2010) Formulation and Evaluation of Torsemide intragastric buoyant sustained release micro particles. J Pharm Res 3(2): 742-746.
- Arya RKK, Singh R, Juya V (2010) Mucoadhesive micro particles of famotidine: Preparation characterization and in vitro Int J Eng Sci Technol 2(6): 1575-1580.
- Cirri M, Maestrelli F, Scuota S, Bazzucchi V, Mura P (2021) Development and microbiological evaluation of chitosan and chitosan-alginate micro particles for vaginal administration of metronidazole. Int J Pharm 598: 1-11.
- Abbaraju KS, Begum N (2015) Biomedical applications of microspheres. J Modern Drug Discov Drug Deliv Res 4(2): 1-5.
- Jayaprakash S, Halith SM, Mohamed Firthouse PU, Kulaturanpillai K (2009) Preparation and evaluation of biodegradable microspheres of methotrexate. Asian J Pharm 3: 26-46.
- Hossain KMZ, Patel U, Ahmed I (2014) Development of microspheres for biomedical applications: A review. Prog Biomater 4(1): 1-19.
- Kedzierewicz F, Thouvenot P, Lemut J, Etienne A, Hoffman M, et al. (1999) Evaluation of peroral silicone dosage forms in humans by gammascintigraphy. J Control Release 58: 195-205.
- Kreuter J, Nefzger M, Liehl E, Czok R, Voges R (1983) Microspheres - A Novel Approach in Drug Delivery System. J Pharm Sci 72: 1146.
- Pradeesh TS, Sunny MC, Varma HK, Ramesh P (2005) Preparation of microstructured hydroxyapatite microspheres using oil in water emulsions. Bull Materials Sci 28: 383-390.